The Many Faces of Diphenylalanine
DOI:
https://doi.org/10.13052/jsame2245-4551.123Keywords:
Diphenylalanine, peptide self-assembly, peptide nanowires, peptide nanotubesAbstract
Diphenylalanine is well known to form complex self-assembled structures,
including peptide nanowires, with morphologies depending on N- and C-
terminal modifications. Here we report that significant morphological vari-
ations of self-assembled structures are attainable through pH variation of
unmodified diphenylalanine in trifluoroethanol. The obtained self-assembled
diphenylalanine nanostructures are found to vary drastically with pH, incu-
bation time, and diphenylalanine concentration in solution. The observed
structures ranged from structured films at neutral and alkaline conditions to
vertically aligned nanowires and sponge-like structures at acidic conditions.
These observations are corroborated by the results of electrostatic modelling,
indicating the disappearance of the dipole moment at high pH values. This
also emphasizes the importance of the dipole moment for the resulting self-
assembled structures. Our results suggest that, in comparison to the commonly
described procedure of diphenylaniline nanowire growth through aniline
vapor treatment, strictly anhydrous conditions are not necessarily required.
Downloads
References
A. M. Kushner and Z. Guan. Modular Design in Natural and Biomimetic Soft Materials.
Angew. Chem. Int. Ed., 50:9026–9057 (2011).
L. Liu, K. Busuttil, S. Zhang, Y. Yang, C. Wang, F. Besenbachera and M. Dong. The role
of self-assembling polypeptides in building nanomaterials. Phys. Chem. Chem. Phys.,
:17435–17444 (2011).
R. de la Rica and H. Matsui. Applications of peptide and protein-based materials in
bionanotechnology. Chem. Soc. Rev., 39:3499–3509 (2010).
N. Srinivasan and S. Kumar. Ordered and disordered proteins as nanomaterial building
blocks. WIREs Nanomed Nanobiotechnol, 4:204–218 (2012).
R. B. Merrifield. Solid Phase Peptide Synthesis. I. The Synthesis of a Tetrapeptide. J. Am.
Chem. Soc., 85(14):2149–2154 (1963).
T. Ganz. Defensins: Antimicrobial peptides of innate immunity. Nature Reviews Immunol-
ogy, 3:710–720 (2003).
E. Guani-Guerra , T. Santos-Mendoza, S. O. Lugo-Reyes and L. M. Teran. Antimicrobial
peptides: General overview and clinical implications in human health and disease. Clinical
Immunology, 135:1–11 (2010).
G. Maroti, A. Kereszt, E. Kondorosi and P. Mergaert. Natural roles of antimicrobial
peptides in microbes, plants and animals. Research in Microbiology, 162(4):363–374
(2011).
M. Zasloff. Antimicrobial peptides of multicellular organisms. Nature, 415:389–395
(2002).
H. Raghuraman and A. Chattopadhyay. Melittin: a Membrane-active Peptide with Diverse
Functions. Biosci Rep, 27:189–223 (2007).
G. Terlau and B. M. Olivera. Conus Venoms:ARich Source of Novel Ion Channel-Targeted
Peptides. Physiol Rev, 84:41–68 (2004).
The Many Faces of Diphenylalanine 205
G. Bhak and Y.-J. Choe and S. R. Paik. Mechanism of amyloidogenesis: nucleation-
dependent fibrillation versus double-concerted fibrillation. BMP reports, 42(9):541–551
(2009).
J. A. Hardy and G. A. Higgins. Alzheimer’s disease: The amyloid cascade hypothesis.
Science, 256:184–185 (1992).
M. Stefani, C. M. Dobson. Protein aggregation and aggregate toxicity: new insights
into protein folding, misfolding diseases and biological evolution. Journal of Molecular
Medicine, 81(11):678–699.
J. W. Kelly. The alternative conformations of amyloidogenic proteins and their multi-step
assembly pathways. Curr. Opin. Struct. Biol., 8(1):101–106 (1998).
B. H. Toyama and J. S. Weissman. Amyloid Structure: Conformational Diversity and
Consequences. Annu. Rev. Biochem., 80:557–85 (2011).
A. T. Petkova, R. D. Leapman, Z. Guo, W.-M. Yau, M. P. Mattson, R. Tycko. Self-
Propagating, Molecular-Level Polymorphism in Alzheimer’s β-Amyloid Fibrils. Science,
:262–265 (2005).
M. Reches and E. Gazit. Casting Metal Nanowires Within Discrete Self-Assembled
Peptide Nanotubes. Science 300:625–627 (2003).
M. Reches and E. Gazit. Controlled patterning of aligned self-assembled peptide
nanotubes. Nature Nanotechnology, 1:195–200 (2006).
J. Ryu and C. B. Park. High-Temperature Self-Assembly of Peptides into Vertically Well-
Aligned Nanowires by Aniline Vapor. Advanced Materials, 20:3754–3758 (2008).
J. Ryu and C. B. Park. Solid-Phase Growth of Nanostructures from Amorphous Peptide
Thin Film: Effect of Water Activity and Temperature. Chemistry of Materials, 20:4284–
(2008).
J. Ryu and C. B. Park. Synthesis of Diphenylalanine/Polyaniline Core/Shell Conducting
Nanowires by Peptide Self-Assembly. Angew Chem Int Edit, 48:4820–4823 (2009).
O. Carny, D. E. Shalev and E. Gazit. Fabrication of Coaxial Metal Nanocables Using a
Self-assembled Peptide Nanotube Scaffold. Nano Letters, 6(8):1594–1597 (2006).
M.B. Larsen, K.B. Andersen, W.E. Svendsen and J. Castillo-Leon. Self-Assembled
Peptide Nanotubes as an Etching Material for the Rapid Fabrication of Silicon Wires.
BioNanoSci., 1:31–37 (2011).
I. De Oliveira Matos and W. A. Alves. Electrochemical Determination of Dopamine Based
on Self-Assembled Peptide Nanostructure. ACS Appl. Mater. Interfaces 3:4437–4443
(2011).
M. Yemini, M. Reches, J. Risphon and E. Gazit. Novel Electrochemical Biosens-
ing Platform Using Self-assembled Peptide Nanotubes. Nano Letters, 5(1):183–186,
J. Yuan, J. Chen, Z. Wu, K. Fang and L. Niu. A NADH biosensor based on diphenylala-
nine peptide/carbon nanotube nanocomposite. Journal of Electroanalytical Chemistry,
:120–124 (2011).
T. J. Dolinsky, J. E. Nielsen, J. A. McCammon and N. A. Baker. PDB2PQR: an auto-
mated pipeline for the setup, execution, and analysis of Poisson-Boltzmann electrostatics
calculations. Nucleic Acids Research, 32:W665-W667 (2004).
M. H. M. Olsson, C. R. Sndergard, M. Rostkowski and J. H. Jensen. PROPKA3: Consis-
tent Treatment of Internal and Surface Residues in Empirical pKa predictions. Journal of
Chemical Theory and Computation, 7(2):525–537 (2011).
M. Hashemi et al.
N. A. Baker, D. Sept, S. Joseph, M. J. Holst and J. A. McCammon. Electrostatics of
nanosystems: application to microtubules and the ribosome. Proc. Natl. Acad. Sci. USA,
:10037–10041 (2001).
J. Ryu and C. B. Park. High Stability of Self-Assembled Peptide Nanowires Against
Thermal, Chemical, and Proteolytic Attacks. Biotechnol Bioeng, 105(2):221–230 (2010).
N. Hendler, N. Sidelman, M. Reches, E. Gazit, Y. Rosenberg and S. Richter. Formation
of Well-Organized Self-Assembled Films from Peptide Nanotubes. Advanced Materials,
:1485–1488 (2007).
L. Gurevich, T. W. Poulsen, O. Z. Andersen, N. L. Kildeby and P. Fojan. PH-dependent
self-assembly of the short Surfactant-like peptide KA6. J. Nanosci. Nanotechnol.,
:7946–7950 (2010).
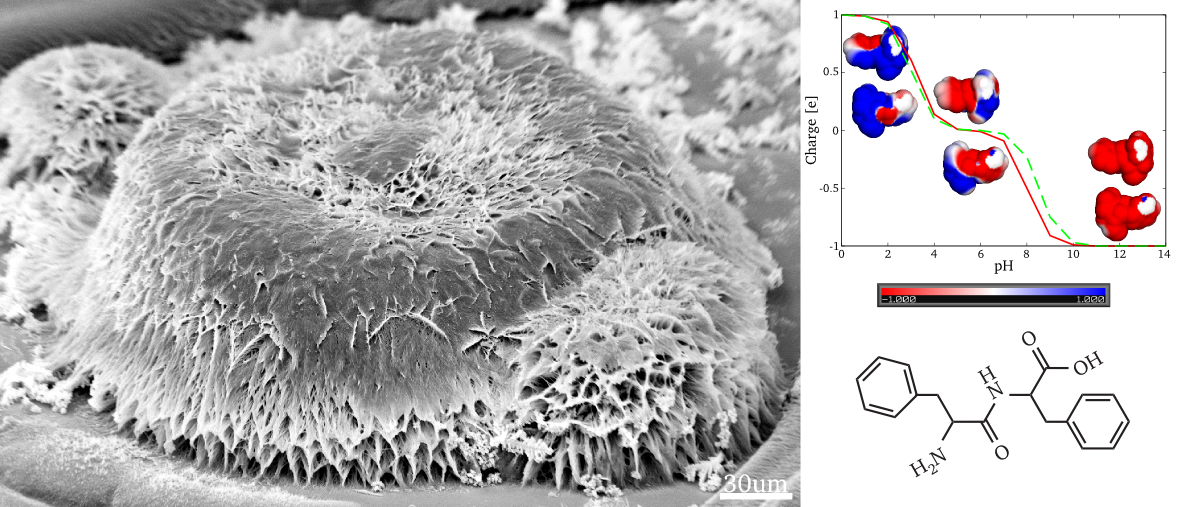